Interstellar Holography
Some radio sources, called "pulsars" (rotating neutron stars), show strong interference fringes: the intensity of the signal at each radio-frequency oscillates on time-scales of seconds to minutes. These oscillations come about because the radio-signals are scattered by lumpiness in the interstellar medium - especially by lumpiness in the ionised interstellar medium - so the received signal is the sum of many waves that have taken different paths from the pulsar to the telescope. This scattering occurs for all radio sources, but most sources are large enough that the oscillations are smeared out and cannot be detected - the interference is constructive for some parts of the source, and destructive for others, with little net effect.
For pulsars, when we record these oscillations for many different radio-frequencies we obtain the "dynamic spectrum", which contains a lot of information on the various scattered waves that are reaching the telescope. It is easiest to visualise the dynamic spectrum by rendering it as an image, with one axis being frequency, the other axis being time, and the brightness of each pixel in the image representing the intensity of the wave at that frequency-and-time. In the simple case where there is one direct and one scattered path to the telescope, the dynamic spectrum might look like this:
In the case shown above we see a single ripple running across the image. The properties of the ripple tell us about the location of the scatterer relative to the location of the pulsar, because (i) waves scattered through large angles cause high-frequency ripples at every instant, and (ii) the rate at which the pulsar is approaching the scatterer (as seen on the sky) determines the rate of rippling in any given radio-frequency channel.
Typically there are large numbers of scattered waves, so the interference pattern appears very complicated, but the principle remains the same: the properties of the scatterers determine the observed dynamic spectrum - it's just that now we need a computer to help us to interpret the observations. An example of a dynamic spectrum arising from 512 scattered waves is shown below.
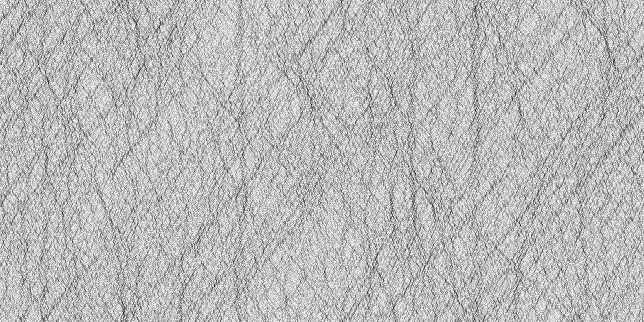
The dynamic spectrum of a pulsar is, in fact, a type of hologram, so this sort of approach to studying the structure of the interstellar medium can be termed "interstellar holography". The key to this technique is being able to determine the contributing scattered waves (E1, E2, E3 …) which make up the pulsar signal: E = E1 + E2 + E3 + … Breaking down the signal in this way is possible because each scattered wave produces a distinct ripple. But it is complicated by the fact that the thing we measure - the dynamic spectrum - is not the signal itself (E, the electric field), but the squared-modulus of th signal |E|2 (the intensity). One way around this problem is to record the dynamic cyclic spectrum of the pulsar.
Once we know the make-up of the pulsar signal in terms of scattered waves, we can proceed to make an image of the interstellar material which has caused the scattering. Early results showed that the scattering material can be almost completely anisotropic - meaning that scattering takes place in one direction but not the other, leading to an image which resembles a line drawn on the sky. That property can be seen with the relatively poor-quality images one obtains in a single observation. By taking observations on many different days we can build-up a picture with much more detail.
We're developing holographic techniques for making detailed images of the interstellar medium using scattered radio waves. These images will help us to resolve some fundamental puzzles about the nature of the material which scatters radio-waves in interstellar space - see the page on interstellar scintillation. A better understanding of radio-wave scattering will also allow assist the various international teams who are aiming to detect low-frequency gravitational waves by measuring pulse arrival times with high precision. Finally, by exploiting the slight differences between the various scattered signals we will be able to obtain pictures of the structure of the radio sources themselves, and that will help us to understand how pulsars emit radio-waves.