Extreme Interstellar Scintillation
Twinkling! When you look up at the night sky you can see the stars twinkle. They do that because of very slight lumpiness in the air, which causes the light to be focused or de-focused as it travels through the Earth's atmosphere. The lumps are moving - carried along with the wind - so the brightness of each star appears to fluctuate rapidly. Using a radio telescope you can also "see" radio stars whose brightness fluctuates in a similar way; the difference is that radio twinkling ("scintillation") is not caused by the Earth's atmosphere, but by lumpiness in the interstellar medium - the very low density gas between the stars. By looking at the way radio sources vary we can learn about the lumpiness of the interstellar medium, particularly the ionised interstellar medium which affects radio-waves much more than neutral gas does.
Radio-wave scintillation is mainly caused by lumps in the size range 10,000 to 100,000,000 km - "size of the Earth" to "size of the Earth's orbit" - these might sound like pretty big lumps, but to astronomers they're small. The image below is a zoom-in of the Hubble Space Telescope picture shown at the top of the page (the Orion Nebula). Even the finest detail of this picture, taken with the best available optical telescope, only shows us features in the interstellar gas down to about 2,000,000,000 km. By studying radio-wave scintillation we can investigate the interstellar gas in much finer detail. Furthermore it doesn't require much ionised gas to affect the propagation of radio-waves, so by using scintillation we have an exquisitely sensitive "microscope" for studying the interstellar medium.

What our "microscope" reveals is mostly what we might expect: structures which are similar to those that we can see on large scales, but smaller. This circumstance is common in nature and is variously referred to as "fractal"/"self-similar"/"scale-invariant" or "power-law". In the interstellar medium it's thought that the scale-invariance is due to turbulence in the interstellar gas. Turbulence is a common phenomenon (it's the cause of the lumpiness in the Earth's atmosphere, for example), so this finding, although important, is not surprising.
But scintillation also shows us that scale-invariance is not the whole story: there's much more structure around one particular size. By chance that size happens to be about the same as the distance of the Earth from the Sun - a distance referred to as one "Astronomical Unit" (1 AU is roughly 150,000,000 km). The most dramatic examples of scintillation on AU scales are known as "Extreme Scattering Events" - one example is shown in the image below. Here a blob of ionised gas, a few AU in size, has crossed the line-of-sight to the radio-source that is being observed. The result is alternate focusing and defocusing, causing large changes in the apparent brightness of the radio source. The duration of the event - about 60 days - coupled with a reasonable guess at the speed, tells us that the size of the blob of ionised gas is a few AU.
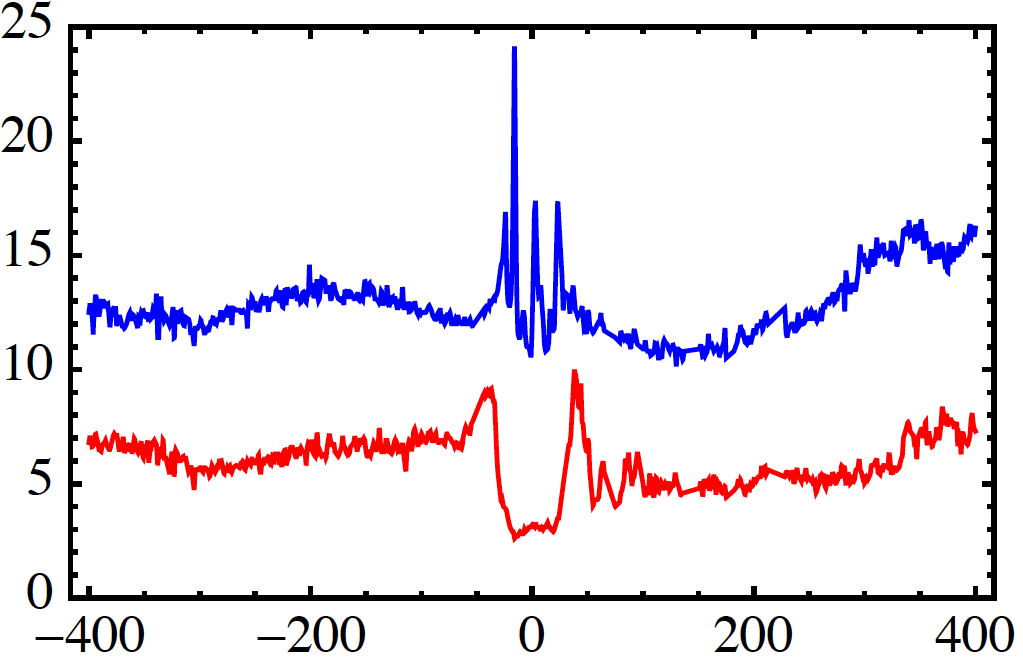
Extreme Scattering Events are well-named: not only do they involve large variations in the apparent brightness of a source, but the blobs of ionised gas which cause these events have enormous pressures. That is very puzzling because it's hard to understand how such high pressures could be maintained - an unconfined gas at very high pressure is, by another name, an explosion, and that's the end of it. This problem would not be much of a worry if we were discussing a rare, freakish sort of event that could be caused by an unusual confluence of circumstances. But the opposite is true. The rate at which Extreme Scattering Events occur tells us that the regions of high-pressure ionised gas are very numerous, with many-thousand times more of them in our Galaxy than there are stars. Whatever the explanation, it needs to be pretty ordinary.
Clues to the physical nature of the blobs of ionised gas can be found by looking at the form of the radio-wave variations in the Extreme Scattering Event shown above. The first thing to notice is that there is a sort of rough symmetry to the event, seen at both high and low radio-frequencies. That's important because - if it's not a fluke - it tells us that the blob of ionised gas which caused the scintillation is itself symmetric in some way. The two illustrations below show examples of the (non)symmetric scintillations which arise from (non)symmetric ionised gas profiles.
The illustrations above show the sort of scintillations which can arise from ionised gas profiles which are or aren't symmetric. On the left is a non-symmetric example, such as might arise from a shock-wave crossing the line-of-sight. On the right is a symmetric example, so the ionised gas distribution on the sky would look like a thin filament. In each case the upper panel shows the amount of ionised gas you're looking through as a function of time, while the lower panel shows how bright the source appears at that time.
For symmetric gas profiles there are only two possibilities: either the ionised gas is mirror-symmetric in one direction, and very extended in the opposite direction, or else it's rotationally symmetric (axisymmetric). But for mirror-symmetric gas profiles a plot of brightness as a function of time should show exactly the same area above the usual brightness level as below. That's not the case for the data (shown earlier), so an axisymmetric distribution of ionised gas is implied.
Assuming, again, that the rough symmetry of the event is not a fluke (a cylinder seen exactly end-on), there is only one three-dimensional shape that can produce the apparent axisymmetry of the ionised gas, i.e. a sphere. Spheres are common in astrophysics - think stars and planets - and it's gravity that's responsible for the sphericity. However, the gravitational force from the ionised gas which focuses the radio waves is insufficient, so we're led to the conclusion that there is a spherical, self-gravitating, neutral gas cloud underlying the ionised gas.
We already have an estimate of the size of the gas cloud (a few AU), and from that we can make a crude estimate of its mass, in the following way. The neutral gas cannot be hot, else we would likely see radiation from it. On the other hand, it cannot be too cold: the Universe is filled with a thermal background radiation of temperature 2.73 degrees Kelvin, left over from the Big Bang, which will warm any matter that is below this temperature. The temperature of a self-gravitating cloud is a gauge of the ratio of its mass to its radius, so if we assume that the cloud is near the cold end of the possible range then we can estimate a mass; the result is a few times the mass of the Earth. These properties don't match anything that we already know about in astronomy. It's convenient to give a name to these "new" objects - on this site we call them "paleons", because it seems likely that they're very old.
Paleons are not things which have previously been recognised and studied as individual entities. They're what astronomers refer to as a type of "dark matter" - matter which is not seen to be emitting light (or other forms of electromagnetic radiation), so its main effect is just the gravity it contributes. Most of the matter in the universe - perhaps 95% of it - is dark matter. When you look at a picture of a galaxy, like the one below, you can see stars and diffuse gas, but there's an awful lot more that you can't see. At present we don't know what the dark matter is made of.
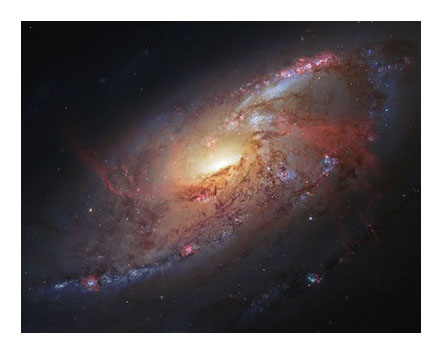
How much of the dark matter could be paleons? The existing data on Extreme Scattering Events are not good enough to give a firm answer to that question. It's possible that the answer could be "all of it" - certainly that has an appealing simplicity to it - but that's an idea that is at odds with the dominant cosmological model, wherein the dark matter is hypothesised to be a new species of elementary particle, usually a Weakly Interacting Massive Particle or "WIMP". Laboratory searches for WIMPs have been undertaken with a variety of carefully designed experiments, but despite the best efforts of many experimental teams around the world, who have pushed technological limits in the process, there have been no convincing WIMP detections to date. The lack of WIMP detections leaves the dominant cosmological model in limbo: it's appealing, but it might not be right.
When studying Extreme Scattering Events one of the key current limitations is that not many of them are known. In April 2014 Manly Astrophysics, in collaboration with CSIRO, initiated a novel project to find new examples of Extreme Scattering Events and study them in detail while they're still underway. This project, named "ATESE", succeeded in finding examples of extreme scattering events. It also discovered examples of another extreme scintillation phenomenon called "Intra-Hour Variability" (IHV), in which the radio brightness of quasars is seen to vary substantially within an interval of an hour.
The IHV phenomenon shares some characteristics with Extreme Scattering Events - high plasma density is required, and the structures that are responsible are small (some 10's of AU), but very numerous - so the two phenomena are likely to have a common cause. A key development in the ATESE project was the identification of IHV in a quasar located very close (on the sky) to the bright star Spica. This discovery prompted us to examine the possibility that IHV is associated with nearby, hot stars; we found that archival data on the IHV phenomenon strongly support that hypothesis, and our own data provided further confirmation.
In coming years we're planning to use a new radio telescope - ASKAP, the Australian Square-Kilometre-Array Pathfinder, which is being built by CSIRO in the West Australian desert - to search for Extreme Scintillation Phenomena. ASKAP is well suited to this task because it can take pictures of a large area of the sky at once, allowing us to search for brightness variations in a huge number of sources.