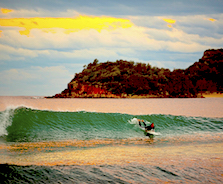
Welcome
Manly Astrophysics is a scientific institute dedicated to research in astronomy.
We focus on understanding astronomical data in terms of the underlying physics.
Our research is described in the "Projects" section, at a level suitable for non-specialists.
News & Views & Events
Manly Astrophysics is excited to share the news that Dr. Willem van Straten, will receive the Astrophysical Software Award 2024 from the German Astronomical Society (Astronomische Gesellschaft, AG) during the next annual meeting of the AG in Köln. This award is presented for the design and development of astrophysical software that has led to remarkable progress in astrophysical research, and Willem is recognized for his significant role in the development of the PSRCHIVE software for pulsar data analysis. As an indication of its impact on the field, the paper that describes the design of PSRCHIVE (Hotan, van Straten, and Manchester 2004) is currently the second-most cited in the Publications of the Astronomical Society of Australia. Willem continues to develop and support this software as part of his research activities at Manly Astrophysics, and he writes about PSRCHIVE’s history and future, below.
I’m honoured to receive the Astrophysical Software Award 2024 from the Astronomische Gesellschaft, and grateful to the many people who contributed to the design and development of the PSRCHIVE software over the years. Since it was first released in 2004, both the functionality and reliability of PSRCHIVE have steadily grown, in large part thanks to the bug reports and suggestions for improvements from the community of people who use the software.
PSRCHIVE evolved out of software that was developed by Matthew Bailes and Jagmit Sandhu to analyse observations made with the Fast Pulsar Timing Machine (FPTM), an autocorrelation spectrometer designed and built at Caltech that was in operation at the Parkes Observatory in the 1990s. When Dick Manchester initiated the development of the PSRFITS file format, we decided to adapt this code such that it could be used to analyse data in either the FPTM or PSRFITS file format. The resulting abstraction made it possible to add support for a variety of file formats, which contributed to the wide-spread adoption of PSRCHIVE by pulsar astronomers around the world.
So what is PSRCHIVE?
PSRCHIVE is open-source software, primarily written in the C++ programming language, that implements a wide range of algorithms for use in pulsar timing, scintillation studies, polarimetry, single-pulse statistics, radio frequency interference mitigation, data visualisation, etc. It is not a single monolithic application with a command language interpreter or integrated graphical user interface, as might be familiar to users of astronomical imaging software. Rather, the most common data analysis tasks are performed using a suite of applications that are designed for command-line execution in a Unix shell. The majority of the functionality compiled into these executables is also available in a library of C++ classes that can be used to develop new applications, and a large fraction of this class library can also be accessed through a Python interface. PSRCHIVE also implements its own domain-specific language that can be used to write small but powerful data processing scripts; these scripts can be executed like a stand-alone application, or passed as arguments to PSRCHIVE applications as pre-processing steps.
But what does PSRCHIVE do?
PSRCHIVE performs many common data processing tasks on pulse profiles, which are averages of some quantity, most often flux density, resolved into some number of pulsar longitude (or phase) bins. It does not perform the time series analysis required to discover new pulsars or produce pulse profiles, such as software packages like SIGPROC, Presto, and DSPSR. The data files input to PSRCHIVE typically contain a three-dimensional array of pulse profiles, in which the total time spanned by the observation is divided into a number of intervals known as sub-integrations, and the observed bandwidth is divided into some number of frequency channels, and at each point in time and frequency, there is a profile for each of the fours Stokes parameters that describe the polarization state of the electromagnetic radiation.
A two-dimensional slice through this four-dimensional data volume is shown in the following plot of the average pulse profile of PSR B1641-45 as a function of pulse phase on the x axis and radio frequency on the y axis.
I like this plot because it demonstrates features of the pulsar, the interstellar medium (ISM), and the instrumentation used to make the observation, all of which feature heavily in my research. On each full rotation of the pulsar, its single narrow beam sweeps over the radio antenna, causing an increase in observed flux density. The ionised component of the ISM causes both dispersive delay and scatter broadening of the pulse, both of which are seen to vary with radio frequency in this plot. The ISM is also the source of the narrow band of additional unpulsed noise near 1420.4 MHz, which is emitted by all of the neutral Hydrogen in our Galaxy along this line of sight. The flux density of the pulsar is strongly attenuated at the edges of the band owing to the filters used to limit the bandwidth of the analog radio signal before it is digitised, and quantization causes the wide band of additional on-pulse noise that is particularly visible at the edges of the band.
PSRCHIVE implements a bunch of fundamental routines for correcting dispersive delays between frequency channels, identifying and excising data that have been corrupted by radio frequency interference, integrating profiles over time and frequency, and estimating the time at which a fiducial phase of the pulsar’s periodic signal arrives at the telescope. Tracking the phase of this signal is the basis of an experimental activity known as high-precision pulsar timing, and is how pulsar astronomers measure the dynamics of binary systems in order to test our understanding of gravitation.
My research in high-precision pulsar timing motivated me to pay careful attention to instrumental calibration, and the majority of the software that I’ve contributed to PSRCHIVE focuses on modelling and correcting the polarimetric response of the observing apparatus. The instrumental response varies with both radio frequency and time, introducing systematic errors in pulse phase estimates that are predicted to be correlated between pulsars. These errors are an additional source of noise that limits the sensitivity of Pulsar Timing Array experiments to the low-frequency stochastic gravitational wave background.
At Manly Astrophysics, I’m collaborating on the development of interstellar holography, a technique that enables us to correct the scatter broadening of pulses caused by multipath propagation in the ISM. We anticipate that this research will lead to new methods that improve the precision with which we can track the phase of the pulsar signal. In analogy to the adaptive optics systems that bring images back into focus after they’ve been blurred by turbulence in our atmosphere, these new techniques will sharpen the experimental sensitivity of Pulsar Timing Arrays to the gravitational waves emitted by supermassive blackhole binary systems. I’m especially excited to apply these new methods when the Square Kilometre Array Observatory comes online in the near future.
WvS 21st August 2024